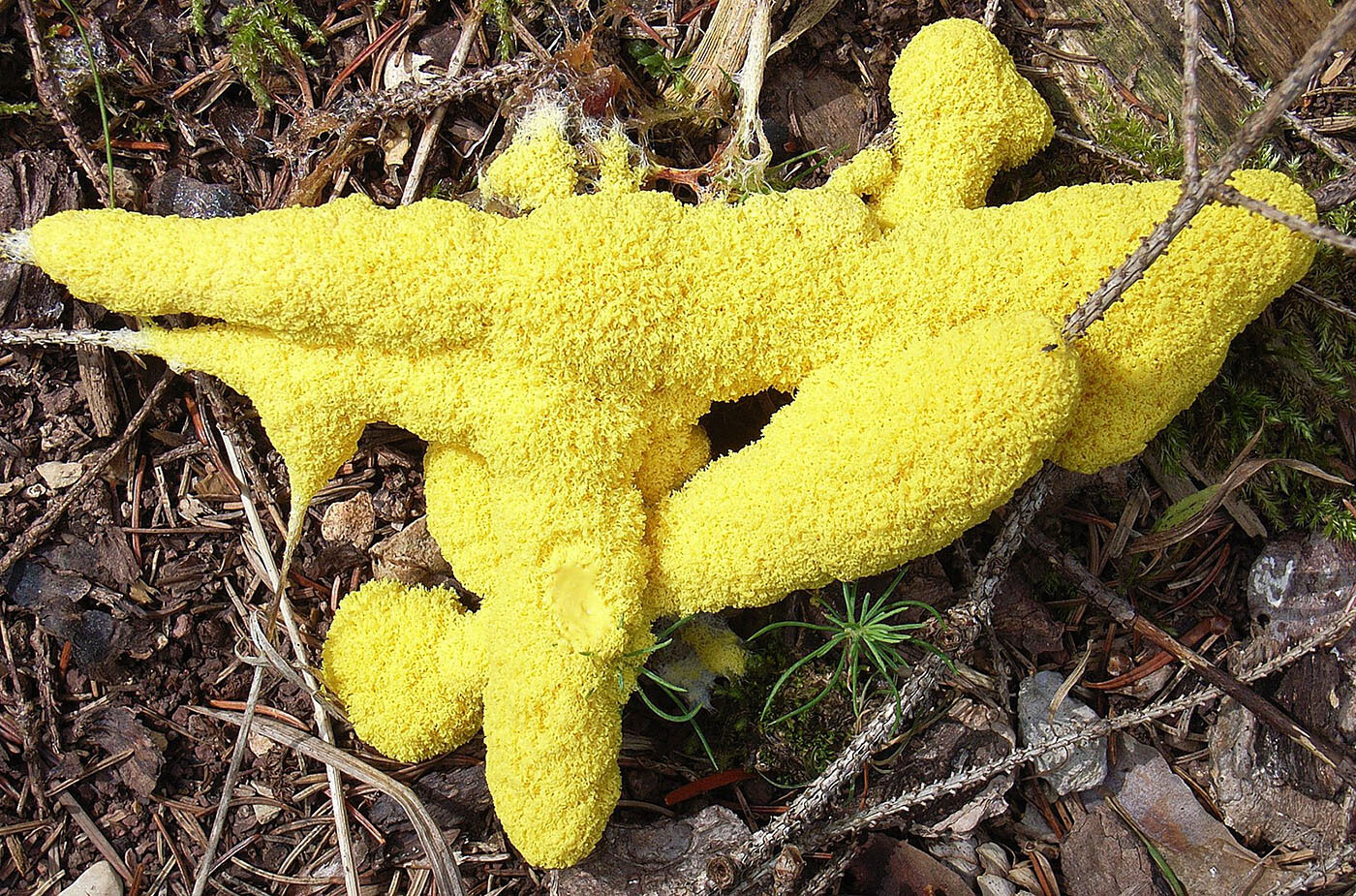
Fuligo septica, the “dog vomit” slime mold. License: public domain.
In Latin, “virus” means “slimy liquid” or “potent juice.” It’s something that flows, amorphous yet powerful. In contemporary science and mainstream media, these connotations have been largely lost. A virus is now a “thing,” a material particle. It has moved from liquidity to solidity.
This move is seductive. “Things” come with an air of stability, manipulability, and a promise of control. In this essay I want to explore the seductive nature of the thing-view of viruses in more depth. What makes this way of talking so powerful? And where does it gain its legitimacy? Part of this exploration will also be to work out (some of) the weaknesses of this thing-view of viruses. When does this view start to show cracks? How does it limit or distort viral theory and practice?
There will not be a single or simple answer to these questions, but I hope to show that there is an often-overlooked yet central feature of thing-talk that underlies much of its seductive force: thinking in terms of “things” allows researchers to place power in a well-defined location. “Things” are not just individual material entities. They are sites of power and action. This creates clear targets on which science can focus its force.
A closer look at the foundations of thing-talk in science unveils a more complex and fragile story, a story in which viruses are in flux, in which power does not have a well-defined seat, and in which thinking in terms of process, cooperation, and energy becomes more important than focusing on things-with-power. This alternative story suggests that a shift is needed in the approach of living and struggling with viruses, a shift in the way power is assigned and used.
1. Virulence
Viruses present humans with various challenges. In many cases they can—depending on place and time—turn out to be useful partners for living systems (see also keyword 7). In other cases, they can become virulent. “Virulence” here refers to the capacity of a microorganism to cause damage or disease to its host.
Developing countermeasures against virulent viruses seems simple in theory and difficult in practice. It seems simple because mainstream science paints a simplified picture of these “things”: viruses are particles with a small DNA- or RNA-based genome, packaged with a few different proteins and, in some cases, a lipid membrane. These are the molecular entities that enter the human body and that infect their cells. These are the particles that are spread when people breathe or sneeze, defecate or bleed.
There are a lot of unknowns in human interactions with viruses, especially when we are facing a newly emerging virus. But the idea of viruses as “things” provides an overall strategy that promises to leave us mainly with known unknowns. Dealing with viral things is about figuring out the internal composition of the entity, both in two dimensions (genome sequence) and in three dimensions (e.g., the structure and arrangement of viral proteins). The idea is that scientists can use the structural information to design drugs or vaccines that target relevant features of viruses. A thing-view of viruses helps biomedical scientists understand the mechanisms these infectious agents use to unfold their power within the host system. Things have particular ways of doing things and the aim of the researcher is to throw a spanner in the works, to physically block the moving parts of those viruses that might pose a danger to the organism’s health.
Where this simple theory becomes difficult in practice is the fact that viruses are highly dynamic. Viruses are the fastest-evolving biological entities, which means that their genetic makeup and structure are constantly changing. As a result, existing drugs or vaccines quickly become outdated; as soon as you know “it” as a thing, the virus has already transformed.
The solution, from the perspective of thing-theory, is to move faster and break the virulent virus before it breaks us. It is a practical issue. It calls for real-time monitoring of circulating viral strains. It demands faster development of vaccines. It is a race between us and them.
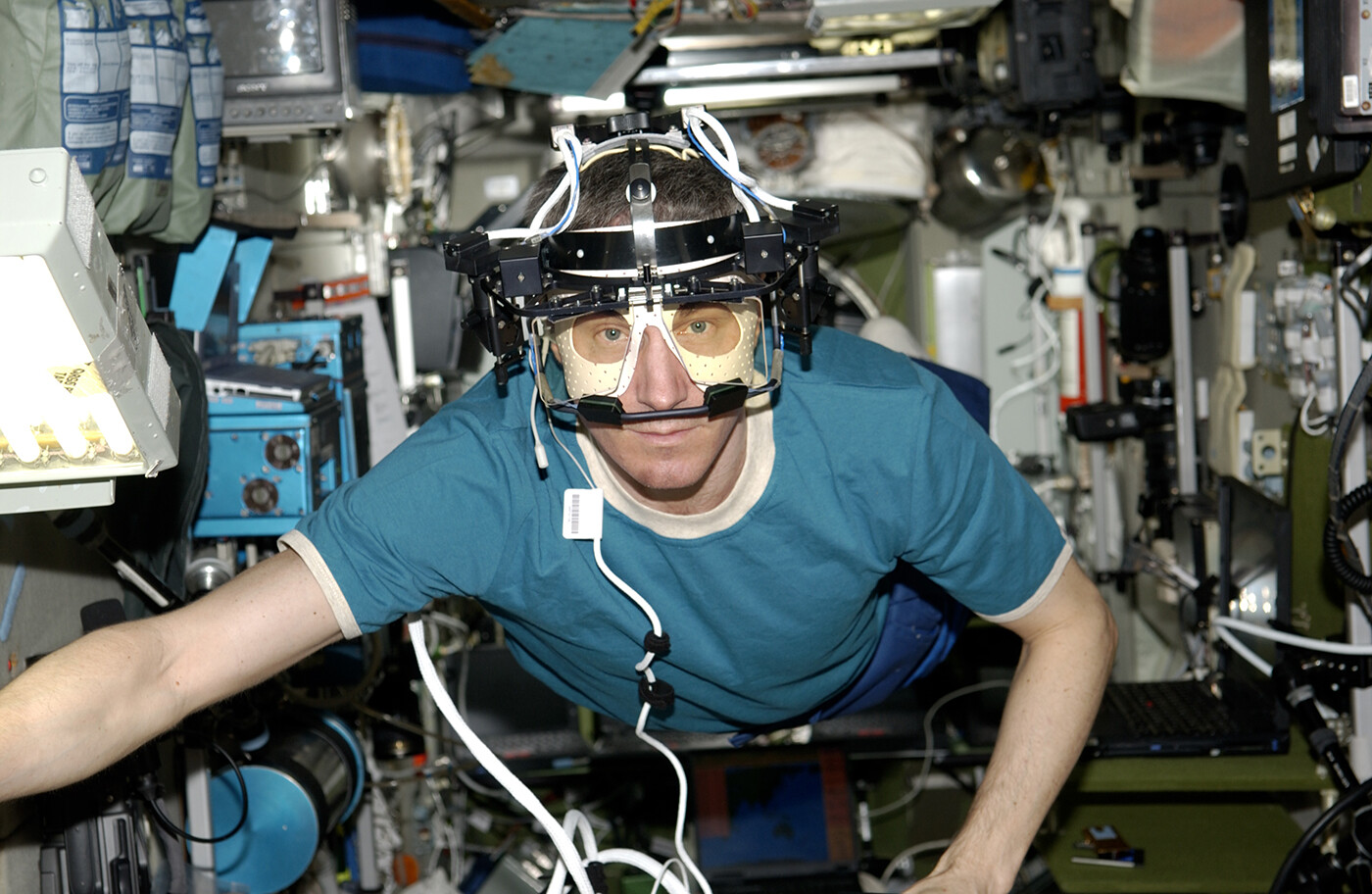
Cosmonaut Sergei Krikalev wears an eye tracking device while on the International Space Station. License: GNU.
2. Hope
At its core, the thing-view is a narrative of hope: even though there are profound uncertainties, there is an overall strategy that can bring humans out on top. We know the enemy and we know how to win the race.
But this cruel optimism comes at a cost. The narrative of scientific hope channels resources and attention in particular ways. It shapes aims, ways of thinking, and which narratives become important. It trains human eyes on technological fixes: tools and machines that can block the activity of the viral thing. A silver bullet against a virus, even if quickly outdated, can be sold for a profit. Techno-optimism calls for fast-tracked drug design and certification, with fewer or shortened tests. It pushes for less scrutiny on government contracts with the private sector, in the interest of time. It shapes who sits at the table when discussing how to respond to a viral threat. It localizes and solidifies power.
Some might argue that this cost is justified because the narrative ultimately delivers solutions that help “everyone.” But this is questionable. Viruses seem to have a way of undermining the quick technological fix. From monkeypox to polio to HIV and coronaviruses, the viral realm is constantly throwing up new surprises and seemingly unpredictable twists and turns. Importantly, these twists and turns inflict pain on people and communities who often don’t get to sit at the table, and whose voices are usually not heard.
The real challenge then is not so much to break viruses with speed, but to develop other hopeful narratives that can be more inclusive, and more open in the way they approach the question of how to live with viruses. Narratives are needed that can make sense of the amorphous and relational power of viruses-as-liquids, instead of thing-viruses. A first step in addressing this challenge is to critically assess the idea of viral “things” that still dominates the debate. Where does this narrative gain its power? Where does it go wrong? And what would an alternative look like?
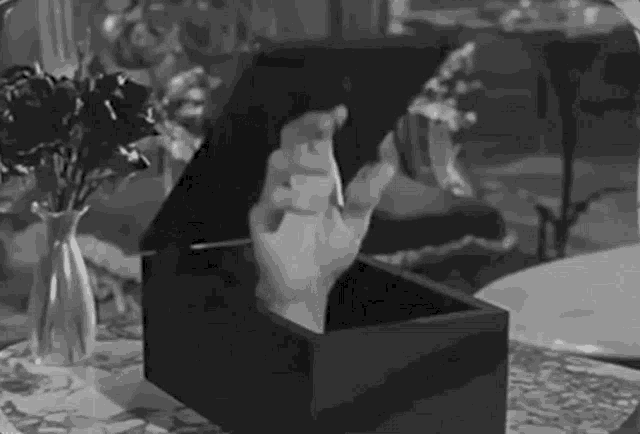
Thing, a fictional character in The Addams Family series. Image: Metro-Goldwyn-Mayer.
3. Things
The thing-view of viruses is closely associated with what philosophers call a “substance ontology.” The term “substance” is a complex technical notion that has received various interpretations in the philosophical literature, going back all the way to Aristotle and the pre-Socratic philosophers. This variety of interpretations causes issues for critical debates about the merits and the downsides of a substance or “thing” view: any critique of such a view is easily fended off by pointing out that the critics are using a flawed, simplistic, or plain wrong understanding of the term “substance.” The debate then meanders into incredibly nuanced but also abstract and long-winded reflections on what this term could or should mean.
Luckily, the mainstream debate about viruses is based on a somewhat simplistic thing-view. At the core of this view are ideas of independence, stability, and power. The independence mainly has to do with how a thing exists in the world: it is usually assumed that a thing has “its” character or identity, independent of what is going on around it. An oxygen atom retains its defining features, independently of whether it is floating through the atmosphere, or whether it is inside a human body. The same for a viral particle.
Connected to this idea of independence is the idea that a thing has certain properties that belong to it and that form its stable core. This matters for how change is understood in a thing-theory: if change happens, it happens to what are assumed to be fundamentally unchanging entities.
Most importantly perhaps, things are also seen as carrying power, a “potential for activity.”1 This translates into them having agency. If something happens, it happens because a thing is doing it. The thing is a locus of action and power.2
Things are usually seen as having their power to do stuff because of their properties, such as microstructure, shape, or mass. This assumption fundamentally shapes how proponents of a thing-view approach viruses and the task of living and struggling with them—the focus is firmly on understanding the microstructure of the viral particle (keyword 2). Knowing its parts and their structure, rather than the broader context or system, allows for a better understanding of what the virus can do, in what situations it will do it, and how researchers can interfere with its functioning. It also defines who or what needs to be mobilized to achieve this goal (experts in structural-material analysis, a pharmaceutical industry that can deliver molecular tools, and so on). Adopting a thing-theory shapes the epistemology, methodology, and politics of human interactions with viruses.
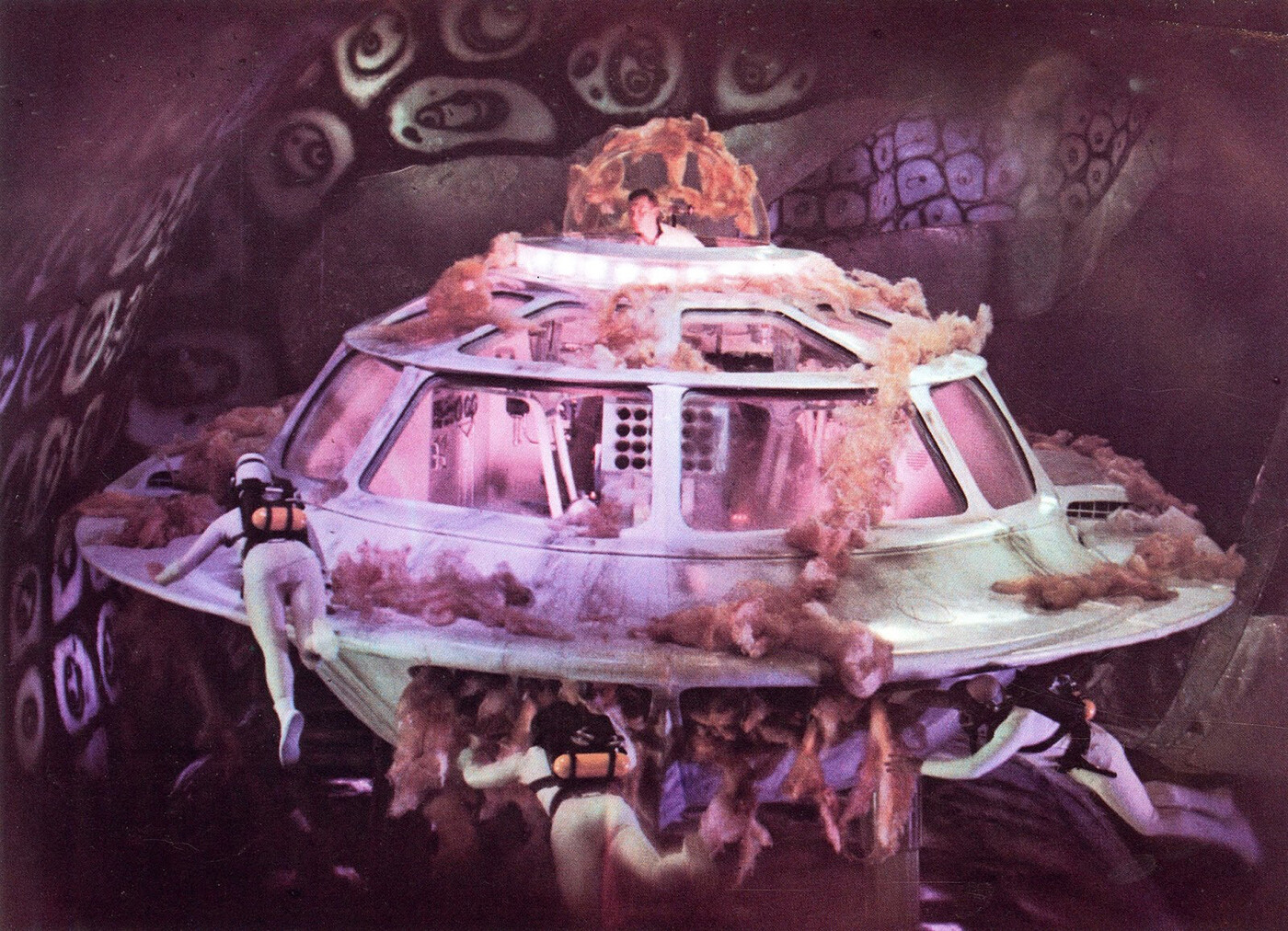
Fantastic Voyage, 1966 (still). Fantastic Voyage is an American science fiction adventure film directed by Richard Fleischer and written by Harry Kleiner, based on a story by Otto Klement and Jerome Bixby. The film is about a submarine crew who are shrunk to microscopic size and venture into the body of an injured scientist to repair damage to his brain.
4. Fictions
Part of the allure of a thing-theory is that it closely aligns with how the natural sciences are speaking. Locating agency in individual things is particularly popular in biology: viruses are said to “jump”; neurons “fire”; gated channels “transport” ions across membranes; regulatory DNA sequences “block” the expression of genes; and so on. This gives the thing-view legitimacy; the authority of science is behind it.
The problem of course is that ways of talking can be misleading. Yes, scientists talk about “things” and what these things can do almost as if they were some sort of human agent. But this does not mean that such a way of talking is more than just a form of convenience, or ignorance, or tradition. Scientists need to communicate with each other and with the public. And they need to package complex ideas into simplifying concepts and models, not only to communicate, but also to learn. Sometimes it might be overlooked that these are simplifications. Their pervasiveness makes them second nature. They become (and come with) practice.3
To give an example: chemists still talk of “the” covalent chemical bond. But it is also true that the bond does not exist in the way it is often depicted and conceptualized. As Philip Ball puts it, the idea of the chemical bond is a convenient fiction that allows the chemical sciences to work in the way they do.4 It is reasonable to assume that biology is also full of such convenient fictions.
An interesting example of such a fiction in biology is the idea that individual genes have power and agency. Scientists used to talk of “the gene for” trait X or Y, and in the history of genetics genes were often seen as the foundation of life, both in the sense of building blocks and also as animating force. This way of talking, which the historian and philosopher of science Evelyn Fox Keller has dubbed “the discourse of gene action,” not only attributes agency but also autonomy to genes.5 Such language presented these entities as some sort of independent causal power that initiates the complex processes underlying living system. This way of viewing genes ignored important molecular interactions and relational aspects of biological systems. It placed power in a very specific and well-defined locus. It is, in many ways, a paradigmatic example of thing-talk at work.
It is well-established now that genes are not “active” in any relevant sense. They are mere strings of nucleotides that can serve as a binding site and template for a variety of proteins. But the discourse of gene action remained an important part of doing science. Keller argued that this dominance was not based on empirical evidence, but rather on the political and cognitive roles that this form of discourse could play within science. Furthermore, according to Keller, it provided researchers with a well-defined locus of action, and this helped them frame the questions they could ask, decide what organisms to use for experiments, which explanations were acceptable, and which weren’t.
The use of a simplistic thing-view of genes was not confined to science. As Kathleen McAfee pointed out two decades ago, a simplistic vision of genes as discrete things with stable and predictable properties has also been mobilized by the biotechnology industry to support a vision of genetic engineering as a precision science.6 This simplistic talk ignored how organisms, genes, and multiple parts of the organism are co-constructed in a dynamic relationship, which includes social and environmental factors. The thing-view allowed governments and private companies to push a neoliberal agenda, in which genetic information could become a commodity that can be patented and traded. Scientific uncertainties and broader socioeconomic factors could be safely ignored. Technologies developed in the Global North could be presented as universal solutions that work independently of context.7
DNA has now been reconceptualized as an inert thing, rather than the locus of thing-power. But while the discourse of gene action has lost importance, the idea of thing-power has not, as the notion of active and powerful things has shifted to proteins. What we end up with is a “discourse of protein action” rather than one of gene action. This new discourse also shapes contemporary virology, much like that of gene action shaped genomics.
5. Proteios
The term “protein” derives from Greek “proteios,” which means “primary” or “of first rank.” Proteins are everywhere in biological systems. They are often described as “the doers” of biology: they digest food, allow signals to be transmitted across cells, or transport molecules across barriers. They are what keeps biological systems going.
The shift from genes to proteins in the discourse of action in biology matters for the debate about viruses. The specific proteins a virus carries, and especially its enzymes, are thought to determine its characteristic behavior. They are primary, or of first rank when it comes to ways of acting: to understand a virus we need to understand the proteins it carries or codes for. Insight comes from looking inside the virus.
To understand this focus on proteins it is important to understand what enzymes are. Enzymes are proteins that function as catalysts, meaning their presence in a reaction system enables a chemical reaction to run faster than an uncatalyzed reaction. The reason a catalyst has this effect is because it allows the reaction to happen with less effort. “Effort” here has to do with energy: most chemical reactions need an initial energy push to get going. For instance, we have to rub the match against a rough surface in order to ignite it (the rubbing creates heat energy). A catalyst opens up a way of getting to the same outcome via a path that does not require as much activation energy. If we had a catalyst for our match, less rubbing would be needed. In some cases, the reaction might even happen spontaneously at room temperature. Without the presence of enzymes most of the processes that organisms rely on could not work at body temperature.
Each enzyme is thought to have “its” particular power. Therefore, depending on what enzymes a virus contains, it can do specific things. The ways of acting are specified by the parts of the virus.
A good example of this is the so-called “retrovirus.” This class of RNA viruses carries a special enzyme that can copy an RNA molecule into a DNA molecule. This is special because turning RNA into DNA violates the “central dogma” of molecular biology, i.e., the idea that genetic information always flows from DNA to RNA, and from there into the production of proteins. When in 1970 two research groups—independently of each other—discovered “reverse transcription,” the dogma was turned upside down.8
Having a reverse transcriptase in their arsenal allows retroviruses to have their own characteristic life cycle. The RNA-based genome of retroviruses can be transcribed into a DNA molecule, and this DNA can then be inserted into the human genome (thanks, again, to special viral proteins called “integrases”). The retroviral genome thus becomes an integral part of the human genome, something that other viruses cannot do because they lack the required proteins.
This narrative of the retrovirus neatly aligns with a thing-view: the characteristic life cycle of the virus is set by its intrinsic capacities. These capacities are defined by the properties of the viral components, in particular the proteins; the viral thing has “its” life cycle program due to the parts it is composed of.
The discourse of protein action thus provides a simple script for how to study viruses and how to design solutions to stop or slow them down. To change viral behavior, we need to block or remove or change its proteins. This is where thing-talk in virology gathers most of its seductive power: it removes fluidity when it comes to the allocation of power. It reaffirms that there is a stable and fixed seat of viral power. It sets a clear target for intervention that is independent of the viral context. The problem is that this simple script, like the discourse of gene action, is undermined by what is now known about how viruses and proteins behave.
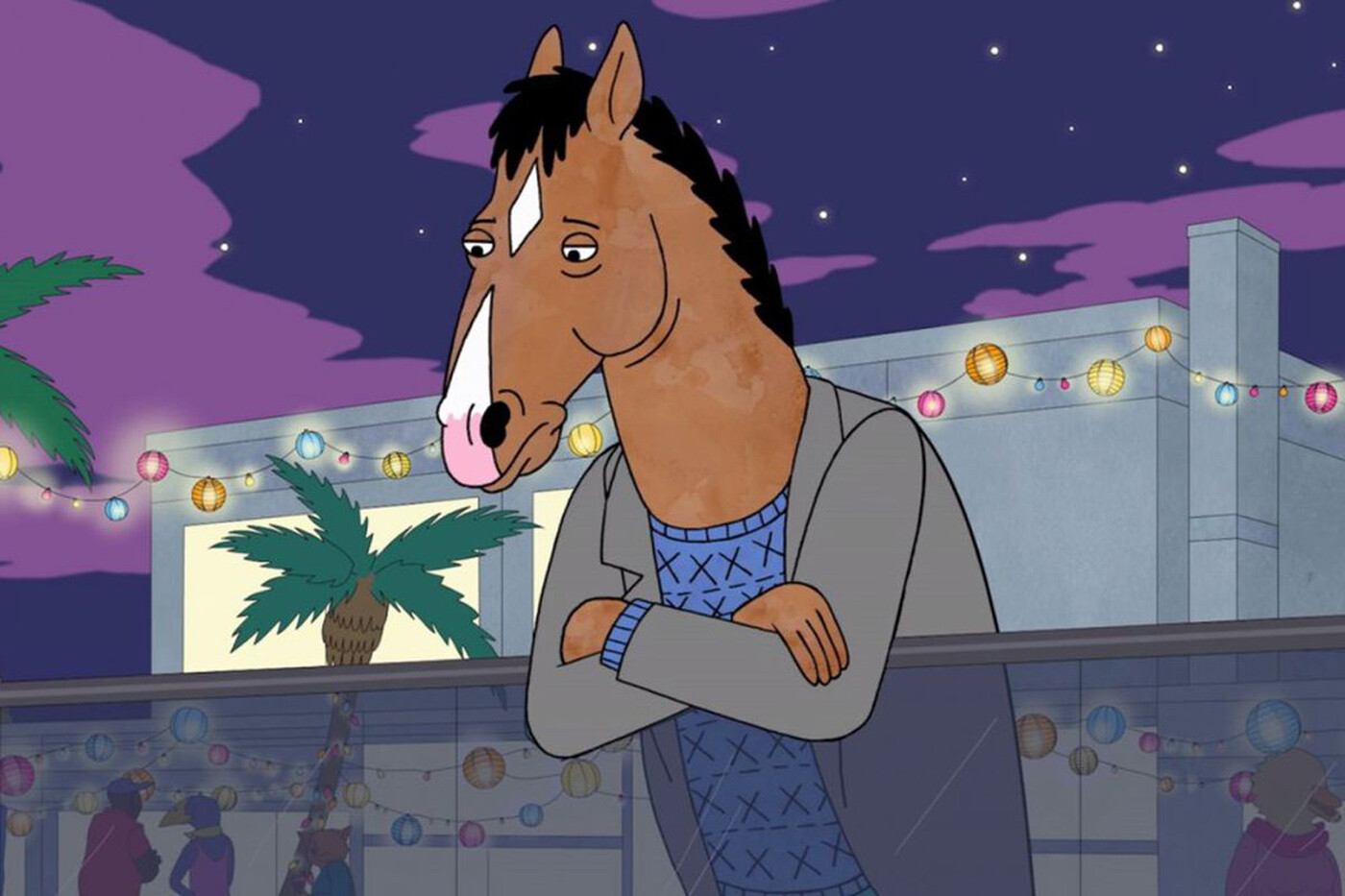
A sad BoJack Horseman leans over a ledge (still from BoJack Horseman). Image: Netflix.
6. Life Cycle
Borna disease was first identified in horses. It is named after a small town in Eastern Germany where, in 1885, 122 horses died of inflammation of the brain.9 The disease had been known anecdotally in Germany since at least the mid-1700s, as horses in the area had repeatedly been described as having a Kopfkrankheit (disease of the head) that would ultimately lead to death. Symptoms of the disease range from lack of coordination to muscle contractions and even signs of severe depression. The latter explains why the disease was sometimes referred to as “sad horse disease.” The mortality rate in horses is high, between 80 and 100 percent.10
In the 1920s it became clear that a virus was responsible for the disease, and in 1990 it was shown that the Borna Disease Virus (BDV) is an enveloped RNA virus.11 Initially identified only in horses, the virus was also found to affect sheep and rabbits. As detection methods further developed it became clear that the virus could infect a number of hosts, ranging from cattle to cats, dogs, alpacas, ostriches, and also humans.12
Interestingly, researchers discovered that BDV is integrated into every human genome. This is surprising because BDV is not a retrovirus and does not contain a reverse transcriptase or integrase. In its “normal” life cycle the virus establishes a persistent infection in the nucleus of host cells, but it does not have the tools to integrate its genome into the host genome. The virus simply should not be there.
It is not known how exactly the virus ended up in the human genome, but it is assumed that host processes in our ancestors interacted with the viral infection process, allowing the viral genome to eventually integrate into the genome.13 BDV turns out to be a very old virus: it is known that this process has been going on for almost one hundred million years.14 It has also been taking place in almost all living things: signs of so-called “endogenous bornavirus-like elements” (EBLs) have not only been identified in mammals but also in snakes and even in the American house spider.15 It is clear that this virus has travelled far and wide. And Borna Disease Virus is not the only RNA virus that has entered such a path. Ebola and Marburg viruses have also been found integrated into the genomes of mammals such as bats or possums, with integration events going back forty million years. Other ancient viruses can be found integrated into the genomes of hosts ranging from plants all the way to fungi and single-celled organisms such as amoeba.16
What we see here, then, is a lack of independence. The path a viral “thing” embarks on is not simply set by its parts. The life cycle emerges from a system of interacting processes that goes beyond the virus. A thing-view cannot easily account for what viruses are doing. There is a fluidity to the paths they take (or which they are channeled into) that undermines the simple narratives presented in keywords 3 and 5.
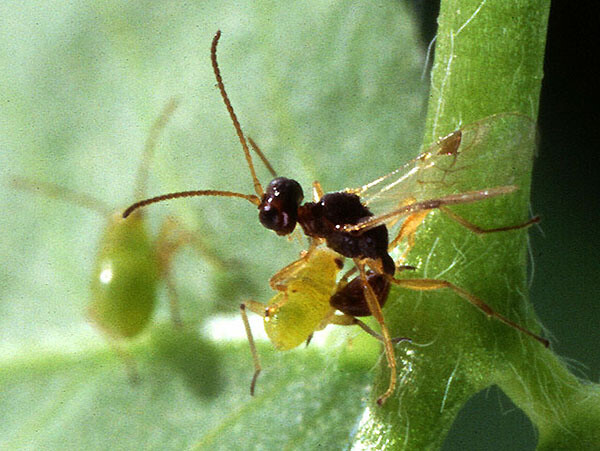
A parasitic wasp, Peristenus digoneutis, prepares to lay an egg in a tarnished plant bug nymph. License: public domain.
7. Pluribiosis
The case of Borna Disease Virus is surprising only from a thing-perspective: it unsettles the conviction that the “active components” of an entity define its potential behavior. BDV moves on several trajectories, which are not some sort of internal program that simply actualizes if the conditions are right. The hostvirus system displays a plurality of ways of behaving. This plurality and fluidity also undermine the narrative of viral pathogenicity, which still dominates how viruses are usually depicted.
Over recent years the pathogen-narrative has started to change, as more and more scientists began to describe viruses as “good” or as potential “friends.” One reason for the emergence of this friend-talk is that researchers uncovered new viruses and new functional connections with organisms, thanks, in part, to novel DNA and RNA sequencing technologies that changed how researchers can detect and analyze viruses. A significant number of these new connections turned out to be mutualistic symbioses, meaning that there is an intimate association between the two (symbiosis) and that both partners benefit from this association (mutualism).
An interesting case of such mutualism—one of many—are parasitic wasps and the viruses they carry. Parasitic wasps have a special life cycle as they lay their eggs in living insect larvae, where they then develop by feeding on the larvae. Insects, however, have developed several defense mechanisms against such parasitic attacks, including the encapsulation of the wasp eggs, which blocks their development. This is where the viral symbiont comes into play: wasps not only inject their eggs, but also viral particles. These particles are produced from viral sequences that have been integrated into the wasp genome over millions of years. The viral particles suppress the larva’s defense mechanisms, allowing the wasp eggs to develop. Both the wasp and the virus therefore benefit from this violent symbiosis that takes advantage of biochemical exploits in the larvae. This example again highlights the heteronomy—rather than the autonomy—of life: the realization of a life cycle is a complex process that depends on several biological activities coming together in a specific manner.17
Talk of “good” or “bad” viruses, however, should not tempt us to split the microbial world into two well-defined parts. As Pierre-Olivier Méthot and Samuel Alizon argue, there is no essence of the pathogen, or the “good” microbe for that matter.18 Microbes are constantly evolving entities that are shaped by their interactions with their host and their ecological context. Recognizing this empirical fact undermines any strict pathogen/nonpathogen distinction. Other dualities such as friend/foe, war/peace, or probiosis/antibiosis also turn out to be too narrow. (“Antibiosis” refers to an interaction between organisms that is detrimental to one of the interaction partners; “probiosis” is the opposite.) Charlotte Brives highlights that these dichotomies still get stuck in a conception of biological entities that emphasizes essence and fixity.19 She introduces the concept of “pluribiosis” to counteract such dualist thinking and to highlight the creative potential of living entities that are always becoming through the interactions they are engaged in. This creative potential or power cannot be easily controlled and subjected to human mastery, as biological systems constantly explore a whole spectrum of relationships (see also keyword 6). There are, consequently, no simple “one-size-fits-all” solutions for intervening in this pluribiotic stream of becoming.20 The manipulability and control promised by the thing-view are likely to fail.
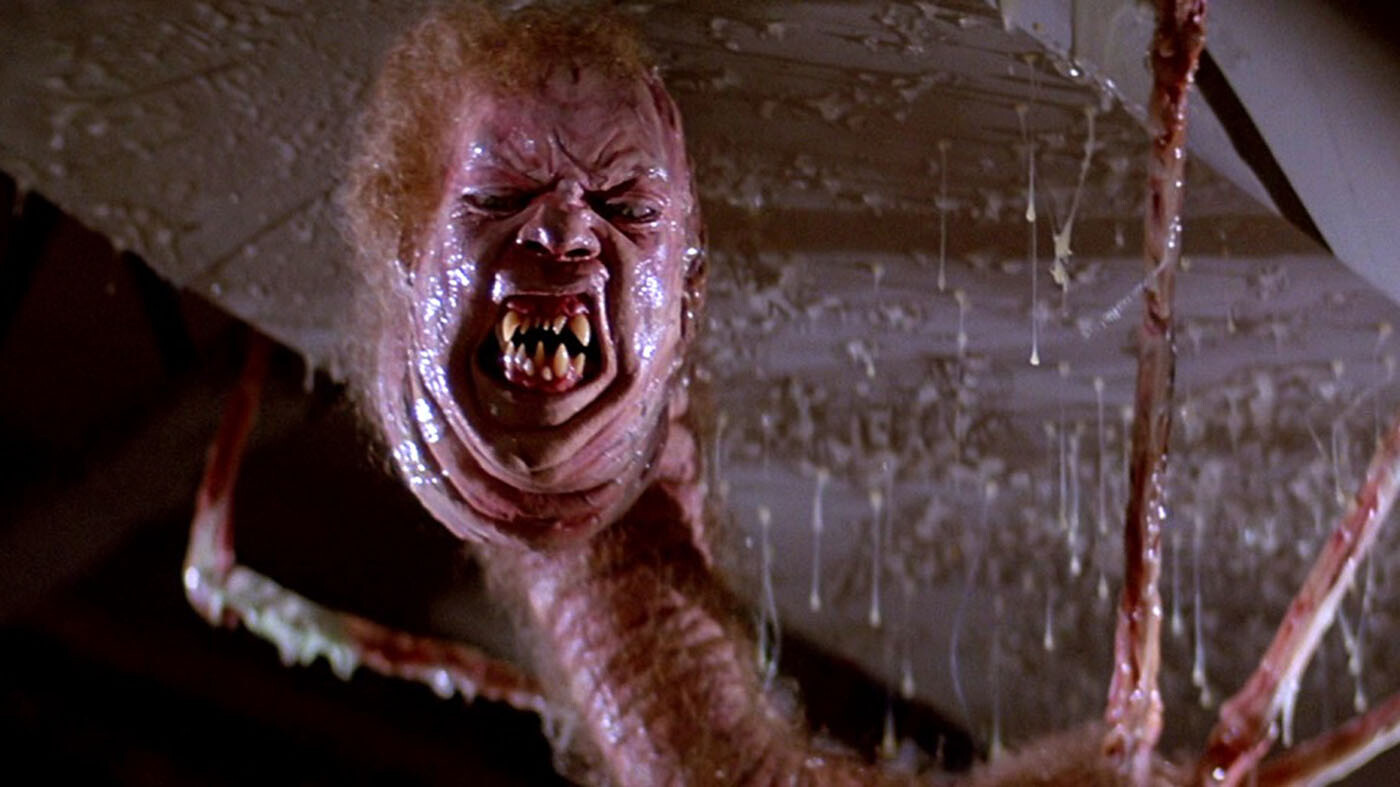
The Thing, 1982, an American science fiction horror film directed by John Carpenter (still). Image: Universal Pictures.
8. Process
The simple thing-view runs into problems when it has to deal with Borna Disease Virus and parasitic wasps. The heteronomy of life and the complexity of life cycles undermine the idea that the properties of a thing somehow decide or prescribe its ways of acting.
But maybe the examples used so far have been unfair. Life and its different cycles are such complex phenomena that it is perhaps little surprise that many different factors and forces are involved in shaping them. So maybe it is necessary to go back to foundational things, such as proteins. This is, after all, where the thing-view seems to unfold its fundamental power. As mentioned above (keyword 5), proteins are the drivers of most processes in living systems. It is here that contemporary biology confidently places power and agency. But where do these drivers get their drive from? What makes them powerful?
In a thing-view the answer to these questions lies in the properties of the protein, and especially its structure and composition. Take the example of enzymes: the reason these entities are powerful drivers of reactions, according to traditional enzymology, is their three-dimensional arrangement; proteins are long chains of chemical building blocks (so-called “amino acids”) that fold into intricate three-dimensional structures. Within these structures, enzymes contain a pocket that has special chemical properties. These pockets, called “active sites”, are special because they offer the chemical reaction an environment in which less energy is required to get from starting point A to product B. The enzyme has power because of its specific microstructure.
However, as the biochemist and philosopher Ross Stein has convincingly argued, this view of enzyme action is highly questionable.21 Building on developments in enzymology, Stein highlights how the “active power” of enzymes is not something that miraculously sits in the protein’s structure. It is rather something that emerges out of the inherent dynamicity of both the protein and its environment. More specifically, the enzyme forms an energetically coupled system with the water molecules surrounding it, and the substrate. It is the integrated movement of this system that ultimately fuels the catalyzed reaction.
For Stein a thing-view can therefore not make sense of the power that enzymes seem to possess. There is not simply a stable and well-defined “thing” that has this power. Whatever it is, the power that researchers observe emerges from a dynamic and deeply interconnected system of processes that forms a distributed field of power and action. What is needed, then, is a view that treats change or activity—rather than being—as fundamental. What is needed, Stein argues, is a process ontology.
A core belief of the views that are subsumed under the label “process ontology” is that physical existence is fundamentally processual. The “things” we encounter, in all their apparent solidity and stability, are ultimately nothing but slow or stabilized processes. As Nicholas Rescher puts it, their permanence is “at best a useful fiction and at worst a misleading delusion.”22 The process view is in many ways a reversal of the thing-view: whereas in the latter activities presuppose the existence of things, the former states that things cannot do without change or activity.
A key motivation for such a reversal comes from the fact that change is ubiquitous in the world. From the erosion of mountains to the flowing of rivers or even political upheaval, the one thing that is constant in the world is change.
But it is also contemporary science that gives strong support for a process view. The development of quantum physics, and especially its challenge to traditional atomism, has, according to Rescher, “put money in the process philosopher’s bank account.”23 And even current biological theory, despite its extensive use of thing-talk (see keyword 4), gives ample support for a process view of biological systems.24 A particularly persuasive piece of evidence is the fact that organisms only exist as long as they are metabolically active: an organism has to maintain a constant flow of matter and energy, otherwise it ceases to be the “thing” it is.25 Becoming is more fundamental than being.
Another aspect of organisms that has been used to motivate a process view of life is the fact that organisms develop; the being of an organism is a constant becoming, as an organism continuously changes its morphology and behavior over the course of its existence. The life cycle is what is basic, and the different stages—the egg, the larva, the adult, etc.—are only time slices of this more fundamental reality.26 The same applies to viruses: what infects an organism is not some sort of well-defined stable “thing,” but a temporally extended viral process.
This last claim might sound strange at first, especially as the idea of the viral particle or “virion” has been burned into our retinas and brains over the last couple of years. Surely this is the virus that infects and threatens us? But a quick look at what a viral infection is will show that the virion, whilst a stage of the viral cycle, is not present during key parts of the infection. In fact, after docking to the target cell, the virion is quickly disintegrated during the entry phase; it is not a permanent feature of the virus. The idea of a “virus,” then, captures a larger entity than just a particle. It captures a whole active cycle, a process which is the virus.27 A process-perspective is a first step away from a narrow thing-view of viruses.
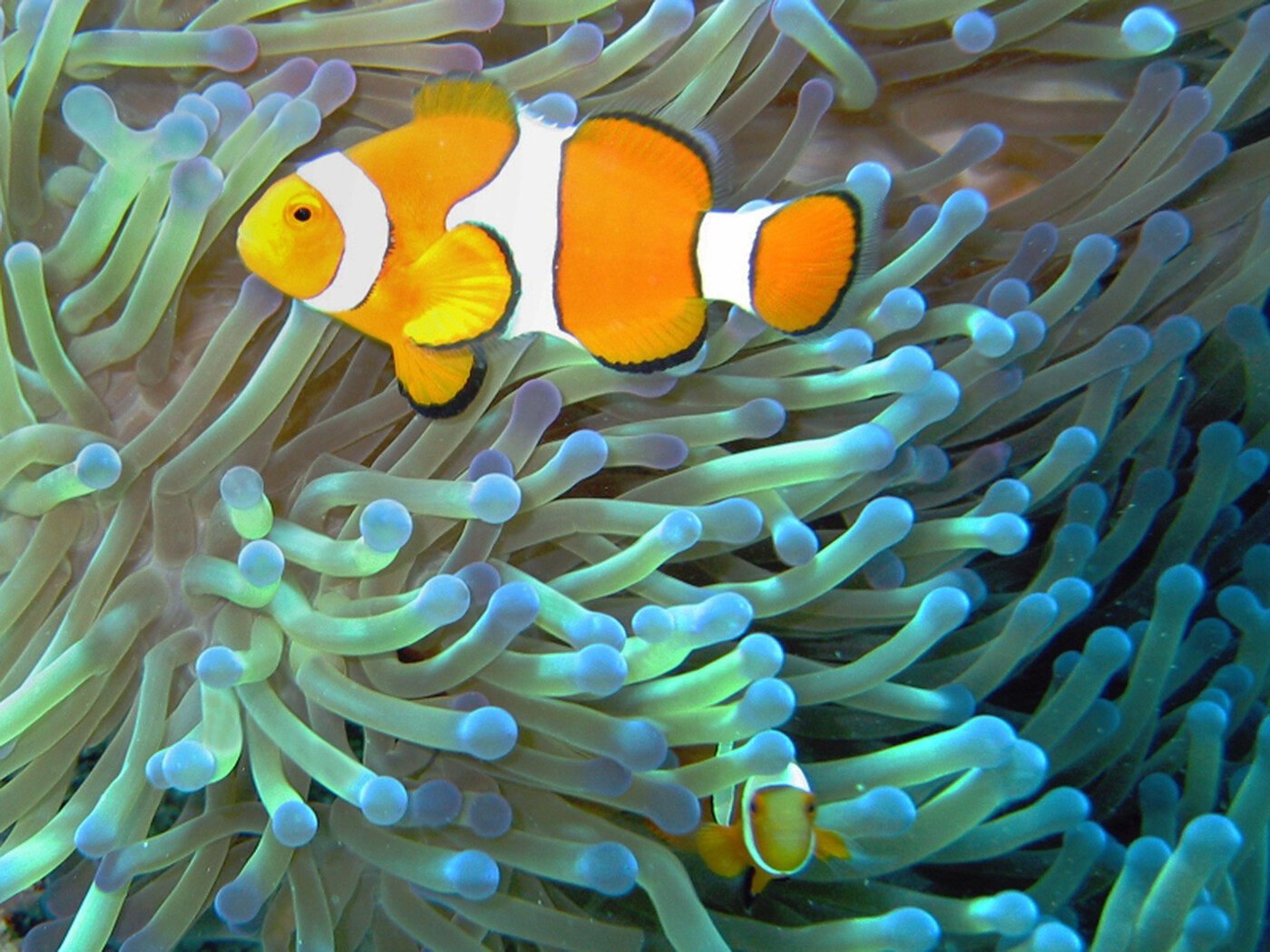
In a cleansing symbiosis, the clownfish feeds on small invertebrates that otherwise have the potential to harm sea anemone, and clownfish fecal matter provides nutrients to the sea anemone. The clownfish is protected from predators by the anemone’s stinging cells, to which the clownfish is immune, and the clownfish emits a high-pitched sound that deters butterfly fish, which would otherwise eat the anemone. License: public domain.
9. Cooperation
The idea of cooperation seems central to both cases described above, and to a process view more generally. In the Borna example, I emphasized that the various life cycles of this virus emerge from a system of interacting processes; the virus could not maintain or realize its life cycles without the cooperation of cellular processes. At the same time, we have also seen that some, or maybe all, organisms could not be the processes they are without the viral process running (the example of parasitic wasps is only one of many examples of virus-host mutualisms). Similarly for the case of enzymes that Stein discusses: there is a dynamic cooperation between the enzyme, the substrate, and the surrounding water.
However, there is a tension that comes with this way of talking. “Cooperation” can be taken to imply that two or more individual agents, each with their own powers, come together to produce a certain outcome. Under such a framing, a key presupposition of a thing-view sneaks back into the idea of “process” view.
Interestingly, the concept of “cooperation” also plays a central role in thing-views that aim to move beyond the simple account described in keyword 3. A good example here is Jane Bennett’s theory of “vibrant matter.” Building on the ideas expressed by proponents of actor-network theory, Bennett proposes that things—and especially material things—have an active, “not-quite-human capaciousness.”28 They have a vitality and power of their own.
This idea of “thing-power” seems to align with the core tenets of the simple thing-view, especially as Bennett, in parts of her writing, talks of things as if they were isolated actors.29 But Bennett also emphasises the need to move away from focusing too much on individuals. She identifies the latent individualism of “thing-power” as one of the key disadvantages of the account. In her view a material thing is always situated within some sort of grouping of things. And it is within such groupings that things gain their power. In fact, things only have power through their cooperation with other things.30 This implies a richer and more complex notion of cooperation than the one implied above.
10. Intra-action
Another way of avoiding the above tension might be to abandon the idea of inter-action altogether. This terminology, after all, presumes the coming-together of two or more preexisting entities or relata. Maybe, then, it would be more apt to approach proteins or viruses from the perspective of Karen Barad’s agential realism and their notion of “intra-action.”31
Agential realism is a relational ontology, and an ontology of becoming. As such it has clear affinities with the ideas that process ontologists are trying to express. Both ontologies abandon the idea of “things” as primary ontological units. Both emphasize the fundamentally active nature of the world, and the primacy of becoming over being. In the agential realism of Barad, well-defined boundaries of the components of a phenomenon are not a given but something that is continuously enacted in ongoing intra-actions. It is not that they simply are determinate; rather they become determinate.32
Barad heavily draws on examples from quantum physics, but the account, perhaps with some modifications, also seems to apply to entities such as proteins. As mentioned above, when we are presented with proteins in scientific discourse, we are usually presented with the idea of well-defined boundaries: each protein has a molecular composition that can be expressed as its “atomic formula” (X amount of carbon atoms, Y oxygen atoms, Z hydrogen atoms, and so on). This makes a protein look like a thing, a particular with clear boundaries and inherent properties.
But if one looks closer at proteins and the practice of science, it becomes evident that these clear boundaries are not a simple given but rather things that emerge from within the intricate controlling and measuring practices that researchers apply in the laboratory. In their handling of proteins, researchers make proteins look like things.33 Stripping away these manipulations, the protein and the water molecules that surround it turn out to be so closely intertwined that it is not always possible to draw a clear boundary between them.34 Whatever the cooperation between water molecules and enzymes, or viruses and cellular processes, amounts to, it does not necessarily have to be cashed out in the sense of inter-action. Using the framework of agential realism to think about viruses seems like a promising strategy to develop a stronger processual alternative to the thing-view of viruses.
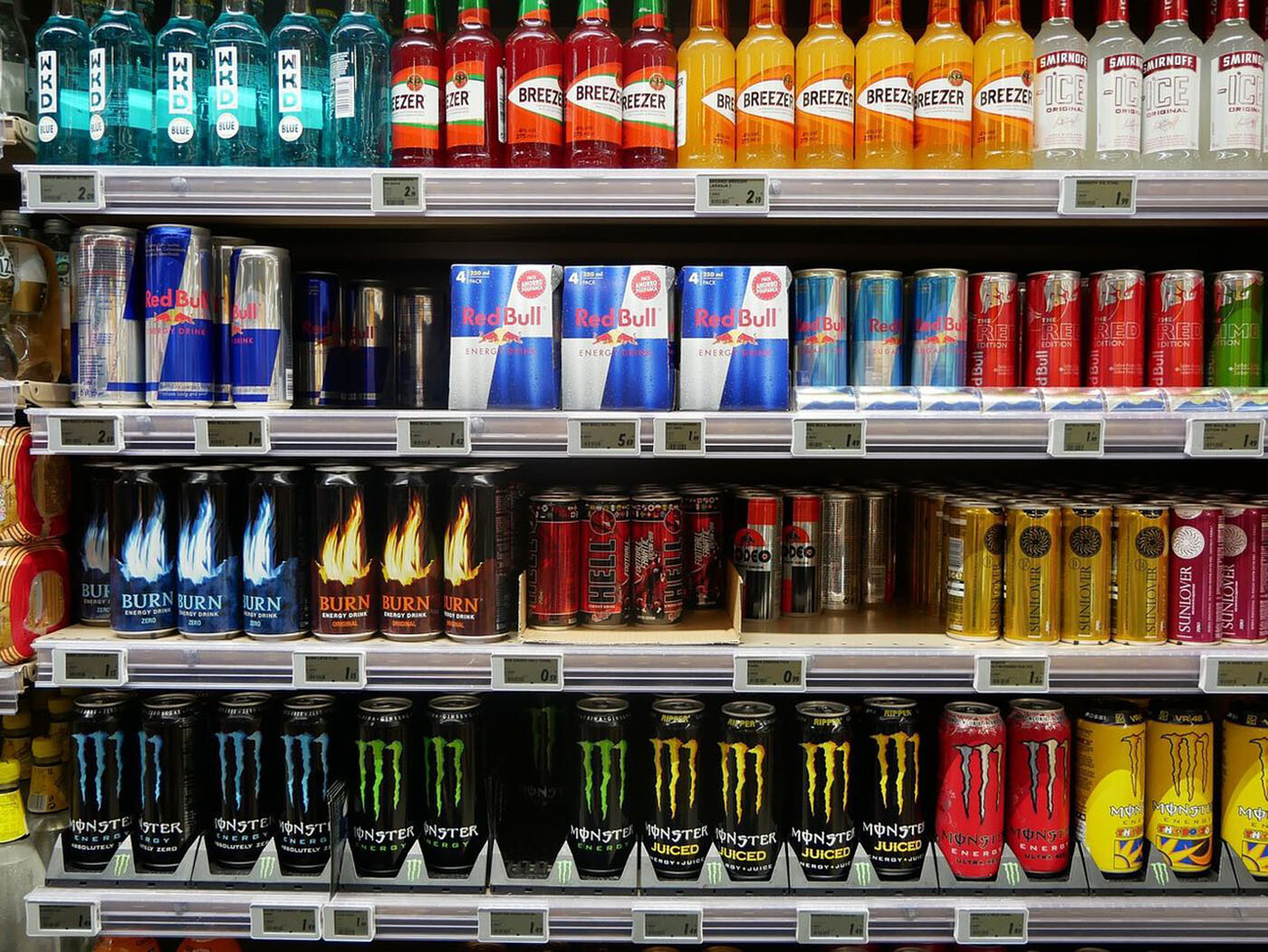
A wide array of energy drinks on display on store shelves.
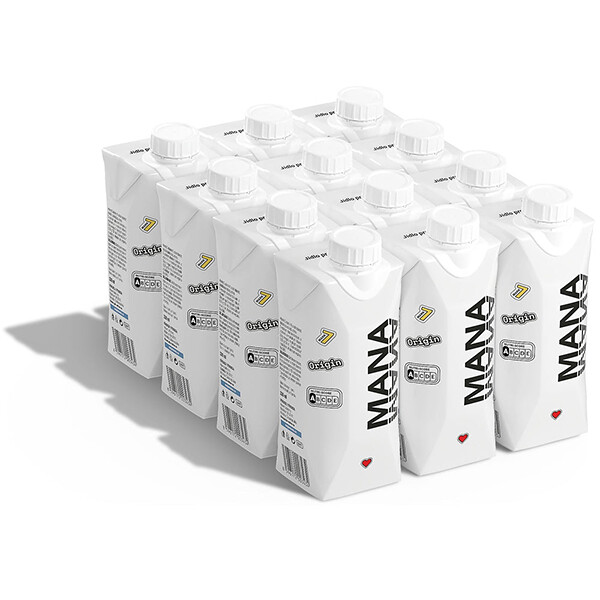
MANA claims to be the seventh iteration of a nutritionally complete food in the form of a drink, with 42 essential nutrients and 330 calories per meal.
A wide array of energy drinks on display on store shelves.
11. Energetics
Maybe the ideas of process and intra-action are enough to move beyond the simple thing-view of viruses. But thinking of “things,” the key issues are not just dynamicity and borders, but also power (keyword 3). A virus is not only amorphous juice, but potent juice.
Where does this viral potency come from? This is the same question asked with regards to enzymes in keyword 8. The answer, looking closely at what Stein is arguing, is not just “process,” but also “energy”: understanding what an enzyme—or a virus for that matter—does means to study flows of energy.35
The concept of “energy,” derived from the Greek word for “activity,” stands for a complex phenomenon that scholars still don’t properly understand. There are useful formalisms in thermodynamics that help make predictions and calculations about physical and chemical processes. But on a metaphysical level, things are less clear. What forms of energy are there? Is there matter that carries energy, or is it all just energy?
A researcher who tried to develop an energetics was Wilhelm Ostwald, one of the founders of physical chemistry and a Nobel Prize winner for chemistry in 1909 (in part for his contributions to the study of catalysis). Ostwald was dissatisfied with the mechanistic worldview that was (and still is) prevalent in science, and a key goal of his work was to overcome scientific materialism. In Ostwald’s view, material bodies were nothing but a spatially copresent complex of energies.36 In this energetics, a piece of matter is not a “carrier” of energy; this would imply that there is more to the object than energy. There are different energies that are coupled and colocalized in these objects. And because they are all energy, they are never independent of the larger system they are in. An energetic view of the world is a fundamentally relational view.
Ostwald’s energetics is riddled with idiosyncrasies and problems. But it might offer a further step forward when thinking about viruses and power. What happens if we view viruses not just through the lenses of process and intra-action, but also through an energetics? What does it mean to define a virus as a complex of energies? I am not sure what the answers to these questions should be. But one conclusion that seems reasonable is that in an energetic view of viruses, power would no longer neatly colocalize with the boundaries of things that we identify by visual and other means. In an energetic view, the power of one area of the energy landscape is inextricably linked to the areas surrounding it; it feeds on and is being fed by the context. Viral particles are temporarily stabilized complexes of energy that emerge from the surrounding energy landscape. Their power is coproduced. And as these fragile powerful flows emerge, they also force other processes, such as organisms and social systems, to spend energy, to save energy, and to change shape. Quite literally, power shifts and is shifted by the process of the virus.
Using words to capture the viral fluidity as a copresence of delocalized powers is difficult. The terminology and formalisms required to properly express a viral energetics are still lacking. Existing notions such as “metabolism” and even the concepts and laws of traditional thermodynamics might not be enough. Writing and speaking about processes also means to constantly fall back on thing-talk, as the English language prefers things. But despite these hurdles, it seems clear that developing an alternative to a thing-view of viruses will require us to not only think about process and intra-action, but also energy.
12. Local
The narrative that emerges from the above examples is one that puts activity before being. It is a narrative that tries to avoid placing power in a specific object. It emphasizes process, intra-action, and energy. It recognizes that science often speaks in a manner that depicts the world as full of active things. But it also shows that no matter from which angle we look, what we find are not things with neatly localized powers, but intertwined flows of energy that give rise to new and complex processes.
This is not to say that proteins or RNA or DNA don’t exist or that they don’t matter. Surely, they are somewhat stable phenomena that we can isolate and manipulate in the laboratory. But they are dynamic stabilities that always emerge in relation to other processes. Much like the idea of the permanence of things, the narrative of independent things with “their” powers is nothing but a sometimes-useful-yet-distorting fiction.
Once we shift the focus away from a narrow thing-view, then the way of thinking about viruses changes. Researchers will still aim to understand their structure, but this structure is not seen as something inherent and as something that bestows potential or power. The virus is a dynamic entity or process that is embedded in, and emerges from, an overarching “energeia,” or activity.
This deeply entangled scenario means that a more integrated, holistic approach to studying and dealing with viruses is needed as compared to the prescriptions of thing-theory. Part of such an integrated approach will be to include knowledge of local context. This context is part of what defines a viral process; the virus in body A is not necessarily the same as the virus in body B, even if it was originally passed on from A to B.
The required solutions are no longer universal technological fixes. Once the simple thing-view of viruses is abandoned, a different way of deploying power emerges. Drugs can help to slow down viral processes in some contexts, and vaccination is a powerful tool to prepare most bodies for the process of infection. But these interventions are only part of the solution. There is a fluidity that underlies the virus-infused world, and once this fluidity is recognized and acknowledged, we can stop thinking about a simple race between us and the virus. The task is no longer to move fast and break things. The task is to understand processes and flows of energies, to understand the creative pluribiotic potential of hostvirus systems and the context they emerge from.
Ruth Porter Groff, “Conceptualizing Causal Powers: Activity, Capacity, Essence, Necessitation,” Synthese 199, no. 3 (December 2021): 9881–96.
Jane Bennett, Vibrant Matter: A Political Ecology of Things (Duke University Press, 2009). Note that while Bennett defends a view of “thing-power,” in important ways her approach is more complex than the simplistic thing-view described here. See also keyword 9.
Stephan Guttinger, “Process and Practice: Understanding the Nature of Molecules,” HYLE: International Journal for Philosophy of Chemistry 27, no. 1 (2021): 47–66.
Phillip Ball, “Beyond the Bond,” Nature 469, no. 7328 (January 5, 2011): 26–28.
Evelyn Fox Keller, Refiguring Life: Metaphors of Twentieth-Century Biology (Columbia University Press, 1996).
Kathleen McAfee, “Neoliberalism on the Molecular Scale: Economic and Genetic Reductionism in Biotechnology Battles,” Geoforum 34, no. 2 (May 2003): 203–19.
We see similar narratives of precision and universality emerge in current discussions about genome editing and “molecular scissors,” such as CRISPR-Cas9. See Eben Kirksey, The Mutant Project: Inside the Global Race to Genetically Modify Humans (Bristol University Press, 2021).
David Baltimore, “Viral RNA-Dependent DNA Polymerase: RNA-Dependent DNA Polymerase in Virions of RNA Tumour Viruses,” Nature 226, no. 5252 (1970): 1209–1211; H. M. Temi and S. Mizutami, “RNA-Dependent DNA Polymerase in Virions of Rous Sarcoma Virus,” Nature 226 (1970): 1211–13.
R. Dürrwald and H. Ludwig, “Borna Disease Virus (BDV), A (Zoonotic?) Worldwide Pathogen: A Review of the History of the Disease and the Virus Infection with Comprehensive Bibliography,” Journal of Veterinary Medicine, Series B 44, no. 1–10 (1997): 147–84.
R. Rott and H. Becht, “Natural and Experimental Borna Disease in Animals,” in Borna Disease (Springer-Verlag, 1995), 17–30.
J. C. de la Torre et al., “Molecular Characterization of the Borna Disease Agent,” Virology 179, no. 2 (December 1990): 853–56.
K. M. Carbone, “Borna Disease Virus and Human Disease,” Clinical Microbiology Reviews 1, no.3 (2001): 513–27.
M. Horie and K. Tomonaga, “Paleovirology of Bornaviruses: What Can Be Learned from Molecular Fossils of Bornaviruses,” Virus Research, no. 262 (2019): 2–9.
J. Kawasaki et al., “100-My History of Bornavirus Infections Hidden in Vertebrate Genomes,” Proceedings of the National Academy of Sciences 118, no. 20 (2021).
C. Gilbert et al., “Endogenous Hepadnaviruses, Bornaviruses and Circoviruses in Snakes,” Proceedings of the Royal Society B: Biological Sciences 281, no. 1791 (2014); M. Horie et al., “Comprehensive Analysis of Endogenous Bornavirus-Like Elements in Eukaryote Genomes,” Philosophical Transactions of the Royal Society B: Biological Sciences 368, no. 1626 (2013).
P. Aiewsakun and A. Katzourakis, “Endogenous Viruses: Connecting Recent and Ancient Viral Evolution,” Virology, no. 479 (2015): 26–37.
T. Pradeu, “Mutualistic Viruses and the Heteronomy of Life,” Studies in History and Philosophy of Science Part C: Studies in History and Philosophy of Biological and Biomedical Sciences, no. 59 (2016): 80–88.
P. O. Méthot and S. Alizon, “What Is a Pathogen? Toward a Process View of Host-Parasite Interactions,” Virulence 5, no. 8 (2014): 775–85.
C. Brives, “Pluribiosis and the Never-Ending Microgeohistories,” in With Microbes, ed. C. Brives, M. Rest, and S. Sariola (Mattering Press, 2021).
On the issue of reification in science see also J. Dupré and S. Leonelli, “Process Epistemology in the COVID-19 Era: Rethinking the Research Process to Avoid Dangerous Forms of Reification,” European Journal for Philosophy of Science 12, no. 1 (2022): 1–22.
R. L. Stein, “Towards a Process Philosophy of Chemistry,” Hyle: International Journal for Philosophy of Chemistry 10, no. 4 (2004): 5–22.
Nicholas Rescher, Process Metaphysics: An Introduction to Process Philosophy (SUNY Press, 1996), 28.
Rescher, Process Metaphysics, 97.
J. Nicholson and John Dupré, Everything Flows: Towards a Processual Philosophy of Biology (Oxford University Press, 2018), 416.
Daniel J. Nicholson, “Reconceptualizing the Organism: From Complex Machine to Flowing Stream,” in Everything Flows, ed. D. J. Nicholson and J. Dupré (Oxford University Press 2018): 139–66.
John Dupré, Processes of Life: Essays in the Philosophy of Biology (Oxford University Press, 2012).
John Dupré and Stephan Guttinger, “Viruses as Living Processes,” Studies in History and Philosophy of Science Part C: Studies in History and Philosophy of Biological and Biomedical Sciences, no. 59 (2016): 109–16.
Bennett, Vibrant Matter, 3.
See S. Abrahamsson et al., “Living with Omega-3: New Materialism and Enduring Concerns,” Environment and Planning D: Society and Space 33, no. 1 (2015): 4–19.
Jane Bennett, “The Force of Things: Steps Toward an Ecology of Matter,” Political Theory 32, no. 3 (2004): 347–72.
Karen Barad, Meeting the Universe Halfway (Duke University Press, 2007).
Barad, Meeting the Universe Halfway, 139.
Guttinger, “Process and Practice.”
M. C. Bellissent-Funel et al., “Water Determines the Structure and Dynamics of Proteins,” Chemical Reviews 116, no. 13 (2016): 7673–97.
The same goes for organisms, which are often described as streams of energy and matter. See Nicholson, “Reconceptualizing the Organism,” 159.
R. J. Deltete, “Wilhelm Ostwald’s energetics 2: energetic theory and applications, part I,” Foundations of Chemistry 9, no. 3 (2007): 265–316.